|
Classification of Landslides
 |
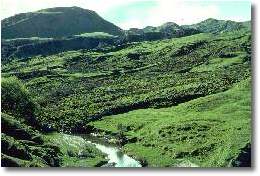
Ngatapa, Tairawhiti
view large image HERE |
|
Any combination of falls, slides and flows can operate in any single
failure. The variety of movements, as well as the immense variation
in landslide materials, mechanisms and rates, all lead to severe difficulties
in formulating a classification of landslides. Much more useful than
arbitrary classification of various forms of slope movements is a
description of the processes involved. The commonly used terminology
is explained in the following notes but it must be realised that all
graduations of processes and materials probably exist particularly
when the type of material which becomes instable is a mixture of rock,
coarse-grained debris and fine-grained soil.
Table 3 shows an abbreviated slope movement classification which identifies
different types of slope movements (e.g. land instability) as (1)
falls, (2) topples, (3) slides, (4) lateral spreads, (5) flows and
(6) complex combinations of the aforementioned. These processes are
illustrated schematically in Figure 2. Table 3.
A classification of landslides (after Varnes 1978).
TYPE OF MOVEMENT |
TYPE
OF MATERIAL |
BEDROCK |
ENGINEERING
SOILS |
Predominantly coarse |
Predominantly fine |
FALLS
|
Rock fall |
Debris fall |
Earth fall |
TOPPLES
|
Rock topple |
Debris topple |
Earth topple |
SLIDES
|
ROTATIONAL |
FEW UNITS |
Rock slump |
Debris slump |
Earth slump |
TRANSLATIONAL |
Rock block slide
Rock slide |
Debris block slide
Debris slide |
Earth block slide
Earth slide |
MANY UNITS |
LATERAL
SPREADS |
Rock spread |
Debris spread |
Earth spread |
FLOWS
|
Rock flow
(deep creep) |
Debris flow |
Earth flow |
(Soil
creep) |
COMPLEX Combination
of two or more principal types of movement |
Figure
2.
Falls
Falls are the simplest kind of landslide movement and are generated
on the steepest hill slopes. They occur where rock discontinuities
such as joints, bedding surfaces and foliation
are relatively closely spaced. Weathering contributes to
the breaking of the rocks along these discontinuities, or clefts,
further weakening them. Water occupying the discontinuities increases
the "cleft-water pressure" and further weakens the clefts
and is responsible for many incidences of rockfall. Once the overall
rock strength is overcome along the cleft the rock shears along
the discontinuity triggering a rockfall. In Norway 60 % of rockfalls
occur in April-May and October-November, which correspond to the
seasons of snowmelt and rainfall maximum. The debris from rockslides
accumulates beneath the cliff as talus or scree or
is carried away by streams (Carson and Kirby, 1972, p. 125-128;
Costa and Baker, 1981, p. 248-249).
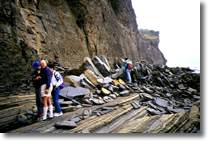 |
 |
An example of a Topple, from Pingchau Island, Hong Kong.
The failure occurred within sedimentary rocks. The orientation
of the rock slabs on the coastal platform indicates failure
of a tall wedge of rock (originating in the rock face
behind the geologists) along a joint plane.
View large image HERE |
|
|
Topples Topples (slab
failures) occur when a slab of rock is pushed outwards along a joint
away from the rock face either by the adjacent rock units or water
or fluid in the cracks between the units of rock. The unit being pushed
rotates forward about a pivot point below or very low in the unit
and creates a wedge of rock that will rotate forward until it eventually
topples over. As it moves forward tension cracks are generated in
the main rock unit and water enters these which then creates joints
and starts the process over again. Although the creeping rotation
of the wedge may be slow once it has moved far enough to overcome
resistance to failure the collapse of the wedge is sudden (Carson
and Kirby, 1972, p. 112-120; Costa and Baker, 1981, p. 251).
An example of a topple occurred when Threatening Rock (New Mexico)
collapsed destroying a portion of the village of Pueblo Bonito. The
village was occupied about 1000 AD. and the hazard that Threatening
Rock posed was recognised then. Then the villagers tried to shore
up the creeping slab of rock. It is calculated that the slab was then
moving about 0.5 mm per year. Before it toppled this rate had increased
to 50 mm per year. Movement accelerated in winter as a result of frost
action and freeze-thaw of water at the base of the slab (Costa &
Baker 1981; Carson and Kirby, 1972, p. 133-134). Slab
and wedge failures are characteristic of well-jointed rocks.
Slab failures occur when sliding takes place along a weakened joint
on an inclined bedding plane. The jointing structure of the rock may
be such that two inclined bedding planes intersect, effectively isolating
a block of rock. If the intersection of these bedding planes dips
towards the face of the cliff face then failure is possible.
Slides
There are two main types of slide, (1) translational slides
and (2) rotational slides (Costa and Baker, p. 251-255)
(1) Translational slides have a relatively flat, planar surface
of movement along one or several surfaces. In block slides
the slide mass moves relatively quickly as a single or few rock
units along steeply dipping bedding or planar joints. In a rock
slide the slide mass is broken into many units. Debris
slides often occur in surficial deposits, i.e. the
loose material which blankets the slope, along a surface of rupture
approximately parallel with the underlying bedrock. The material
may slide as a coherent mass or may break into smaller parts.
With increasing movement downslope debris slides break apart more
rapidly causing the mass to tumble downslope like a snow avalanche.
The momentum of the debris mixture allows it to run out beyond
the foot of the slope. These events, which can be the most hazardous
of all mass movements are called debris avalanches.
(2) Rotational slides have a curved surface of rupture and
produce slumps by their backward rotational movement. Many
landslides that begin with slumping at their main scarp will often
transform to sliding or flowage further downslope. Rotational
slides are typically deep-seated and occur in relatively homogeneous
material and the failure plane often assumes a semi-circular shape.
The 1963 Vaiont Dam disaster, Italy , was caused by a huge 2 km
long, 1.6 km wide, 150 m thick and 240 million m3 rockslide
that displaced water in the reservoir impounded by the Vaiont Dam.
This landslide filled the reservoir for 2 km upstream of the dam
and caused a huge surge wave that travelled across the reservoir,
overtopped the dam and created a 70 m flood wave in the valley below
that killed 3,000 people.
Spreads
Spreading failures occur when sediment (rock or soil) suddenly
loses strength and starts to flow. This process is termed liquefaction.
If the sediment lies beneath firmer rock or soil then the overlying
material is broken up and spreads too. Typically this phenomenon
occurs in very fine-grained sediments and in particular in glacial
quick clays. These clays are very sensitive and have considerable
strength when in an undisturbed condition. However, if disturbed,
e.g. by earthquakes or explosions, they become "quick" and can flow
on slopes as shallow as 1o (Costa and Baker, 1981, p.
255-258)
Soil comprises grains which are in contact with one another. Below
the water table the spaces (voids) between these grains are filled
with water. As long as the grains stay in contact the soil will
retain its strength. However, if pressure exceeding the weight of
the soil is put upon the water in the voids the soil dilates, and
the soil turns into a slurry which behaves like a fluid and has
virtually no bearing strength. This process can happen very quickly
and structures built on top of soil which behaves in this way will
sink into the slurry.
Liquefaction can be triggered by earthquakes (see study guide 4).
Earthquake waves disrupt and reorganise the soil particles which
decreases void space and thus increases pore pressure with pressure
increasing with each passing shock. The size of the grains in the
soil is an important factor. Fine-grained soils, with a large proportion
of silt and clay, are less likely to liquefy because the capillary
water tension between gains is high. Fine- to medium-grained sands
do not allow rapid draining of pore water but have void spaces large
enough to make capillary cohesion irrelevant. These sand sizes are
common in recently deposited marine and river sediments, and so
liquefaction commonly occurs in these materials during earthquakes.
Although liquefaction generally occurs in water-saturated soils,
it can also involve air. During the 16 December 1920 Kansu earthquake,
200,000 people were buried by landslides triggered by liquefaction
of fine-grained loess. The shear strength of the loess was exceeded
by the earthquake effects but low permeability of the fine-grained
soil prevented air from escaping from the loess, which then liquefied.
Quickclays in the St. Lawrence lowland of Canada, the Anchorage
area in Alaska and Scandinavian countries accumulated in marine
coastal areas during glaciations when large quantities of clay was
generated by glaciers grinding down rock inland which was then washed
downstream and into the sea. The salt water caused the clay particles
to bind together in a house of cards fashion with chemicals in the
water providing a glue which created a very strong bond between
the clay particles. Water filled the spaces between the clay particles.
These clays accumulated on the seafloor. As the glaciers melted
receded and their weight stopped pressing down on the land the land
rebounded and raised the muds above sea level. Freshwater then washed
the chemicals (the glue) out of the clays leaving them in unstable
state. When they are shaken, usually during earthquakes, the "house
of cards" structure collapses, the water is released and the
clay flows.
Quick clays also occur in freshwater glacial clays. Here small electrical
forces hold the clays together, rather than a chemical glue. Shaking
of the clay, e.g. during earthquakes, overcomes these forces and
the sediment liquefies.
Debris flows
Most slope failures involve only small movements along the shear
plane before stability is regained and sliding ceases. Thus movement
is generally confined to the limits of the unstable slope. However
landslide debris can become very mobile after slipping to the extent
that debris and contained water is mixed together and then flows
downslope as a fluid, and this is termed a debris flow. Debris
flows can also be triggered by melting snow or rainfall saturated
loose material on hillslopes which then pours downslope. Debris
flows move downslope at a few kilometres an hour, leaving low ridges
of material, or levees, at their margins (Stratham, 1977, p. 93).
They contain 20 to 80 % particles coarser than sand and generally
flow very quickly and are destructive (Costa and Baker, 1981, p.
258)
Debris flows tend to flow down preexisting channels and their movement
resembles wet concrete, and the front of the flow is usually armoured
with boulders which are pushed along in front of the flow. Debris
flows usually contain fine-grained soil material, and this gives
the flow cohesion and allows it to transport very coarse material.
The velocity of the debris flow depends on the thickness of the
flow; once this falls below a critical value flow ceases completely
and the material is rapidly. Plug flow can also occur in
debris flows. In this situation only material at the margins of
the flow is moving and material in the centre of the flow, which
may include cobbles and boulders, is rafted along as a coherent
plug (Costa and Baker, 1981, p. 258-260; Fig. 2).
Many of the most destructive debris flows occur after heavy rainfall
on hillslopes denuded by deforestation (e.g. through forest clearance
or fire). They are generally associated with debris slides and debris
avalanches. A spectacular occurred along the Serra das Araras escarpment,
Brazil, in 1967 when heavy rain triggered hundreds of debris slides
along partings between crystalline bedrock and the overlying soil.
Soil and rock slid into the valley floors, cutting the main highway
between Rio de Janeiro to Sao Paulo, and badly damaging a hydroelectric
complex that supplied power to most of Rio de Janeiro (Costa and
Baker, 1981, p. 261-262).
Creep
In contrast to the processes described so far some mass movements
proceed at rates that are barely detectable. Creep is the
imperceptibly slow down slope movement of regolith (soil and weathered
rock). On much of the New Zealand hill country evidence of creep
can be seen in the formation of small terraces spaced at vertical
intervals of less than a metre which follow the contours of the
hillsides Factors which contribute to creep include frost heaving,
wetting and drying, animal activity and the growth and decay of
plants (Carson and Kirby, 1972, p. 173-176).
|
|