|
Impacts of Wildfires
Fire, either wild or prescribed (a planned fire event), may have a wide range of effects on the bio-geo and atmospheric resources of the area. The wide range of effects is due to the inherent pre-burn variability of these resources, and wildfire behaviour characteristics, season of burning, as well as pre-fire and post-fire environmental conditions such as timing, duration and amount of rainfall. Five systems are of interest: ecosystems (flora and fauna), geosystems (soil and water), the atmosphere, fire management, and society, will be discussed in turn.
Effects on ecosystems
Fire effects on terrestrial wildlife and their habitats as well as plant response to wildfires is addressed in this section.
Plants
The response of plants to fire can vary significantly among fires and on different areas of the same fire. Both variability in the heat regime of the fire and differences in plant species’ abilities to respond affect the post-fire outcome. Fire intensity, burn severity, total duration of combustion, soil heating, time of the year, and time since the last fire, all influence mortality or survival of the plants, and thus subsequent recovery. Post-fire effects also depend on the characteristics of the plant species on the site, their ability to resist the heat of a fire, and the mechanisms by which they recover after the fire (Miller and Findley, 2001). Plant recovery can be affected by factors that vary with the growing season, or age of the plant. Whether the colonising species that first appear after a fire successfully re-establish on the site can be influenced by external factors such as post-fire weather, animal use, and plant competition.
The inherent abilities of plants to respond to fire depend partially on the fire regime to which the plant community has adapted. For example, a plant community may characteristically have been subjected to frequent low intensity, low severity, understorey fires. Knowing the “natural” role of fire on a site gives an indication of the type of plant adaptations to fire that may be present within an ecosystem.
Fire damage to plants
Fire effects on plants include total plant mortality, crown, stem, and/or root mortality. Fire-related tissue mortality is dependant upon both the temperature reached and the duration of time it is exposed to that temperature. Trees can lose 20 to 30% of their crown to fire before the loss affects their growth rate (Fuller, 1991). The lowest temperature at which plant cells die is between about 50 and 55º C, however some are able to withstand exposure to 60º C for a few seconds (Miller and Findley, 2001). Some plant tissue, particularly growing points (meristems or buds) tend to be much more sensitive to heat when they are actively growing, or when their tissue moisture is high. Thus, plant tissues that are actively growing have much higher mortality rates than physiologically dormant tissues or those that have finished active growth for the year. Trees and shrubs can be killed by lethally heating the cambium (Fig. 6), the active growth layer that lies beneath the bark. Bark surface texture and thickness can affect its likelihood of ignition, whether stringy and flammable, or smooth. As with tree and shrub crowns and stems, there are physical and structural characteristics of roots that make them more susceptible to fire damage. Structural support roots growing laterally near the surface are more susceptible to fire damage and consumption than those growing downward. Roots found in organic layers are more likely to be lethally heated than those in mineral soil layers (Miller and Findley, 2001).
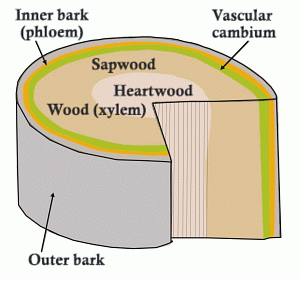
Figure 6. The internal structure of a tree showing the position
of the cambium.
Heating of this active growth layer proves lethal during a wildfire.
Plant mortality depends on the percentage of tissue killed, the location of the dead tissue, reproductive mechanisms, and species ability to recover from injury. Mortality may not occur for several years, and may often be the result of secondary infection by disease, fungus, or insects, because the resistance of plants to these agents is lowered by fire injury, with wound sites providing the entry point for pathogens (Fig. 7) (Littke and Gara, 1986).
Figure 7. Tree roots showing fire injury
(Lenhard, Gerald. Louisiana State University, www.forestryimages.org).
Post-fire plant recovery and growth
Fire causes some important changes in forest systems. It creates openings in the forest, and converts mineral-storing parts of the plants (the stems, leaves, and bark etc) to ash (Fig. 8). In ash form, most of these essential mineral nutrients are dissolved by rain or snow and returned to the soil, to be recycled for further use.
After a fire, herbaceous plants, shrubs, and trees return in a sequence, termed a floral (or seral) succession, with the herbaceous plants arriving first, then the shrubs, and lastly the trees. The plants that return have survived the fire, either by sprouting or from seeds that are already at the site or were carried in. Disturbances like wildfires cause gaps in the forest canopy, creating a mosaic of different stages of succession. The first plants to return are pioneer plants (Fig. 9). They are often weeds or grasses. Next are the seral stage plants, shrubs and trees, which theoretically lead to a stable forest known as a climax forest.
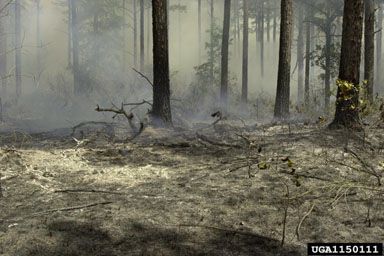
Figure 8. Ash layer blanketing the soil post-fire. The nutrients stored in
this layer will be utilised by the first stage of succession, the pioneer plants
(Lenhard, Gerald, www.forestryimages.org).
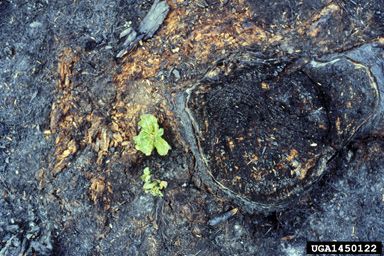
Figure 9. An example of the first stage of post-fire floral succession – re-establishment of pioneer plants (Shorthouse, David, University of Alberta, www.forestryimages.org).
Re-establishment of vegetation after a fire is influenced by various factors, including post-fire weather, the availability of carbohydrates and nitrogen for plant regrowth, post-fire plant competition, and animal use.
Plants that are killed by a fire may persist on the site if they possess adaptive mechanisms that allow the species to persist postfire. Sprouting is one such mechanism that allows plants to recover after a fire. Live shoots can originate from aboveground growing plants or from various depths within the litter, humus, and mineral soil layers. Fire and other disturbances can stimulate sprouting in some plant species through alteration of hormone balances.
Flowering plants successfully establish themselves on burned areas, flourishing in full sunlight and thriving in the lack of competition from other vegetation. Some seeds and pine cones are fire-dependant (such as Pinus. contorta (Fig. 10), and P. banksia) and will not germinate or open without application of heat (which removes seed coatings that inhibit germination).
Figure 10. Pinus contorta
(Mosquin, Daneil, www.ubcbotanicalgarden.org).
Effects on terrestrial wildlife and habitat
No fire – either wild or prescribed – is uniformly “good” or “bad” on the ecosystems involved, with some components responding positively, and others negatively to the stress associated with fire. The effects of fire on animals change over time, but the biggest impact is the modification of their habitat. Few studies have examined the adaptation of animals to fire, but biologists believe that animals with flexible habits and diets (termed generalists) thrive after fires and that those animals that eat foods found only in mature forests (termed specialists) seldom survive (Fuller, 1991).
Much of the impacts of fire on wildlife and wildlife habitat revolve around successional theory, and therefore it is important to understand this concept. Floral succession is one of the primary descriptors of any ecosystem, and refers to the orderly progression of occupancy by successively higher ecological plant communities (Anderson, 2001). This progression from a “pioneer stage” through several intermediate stages to a “climax” (Fig. 11) floral assemblage is often regarded as an overly simplistic view of a highly complex system, whose “orderliness” is often disrupted or altered by events which can neither be anticipated nor controlled. Wildfire is one such event. Short fire intervals tend to promote early successional conditions, such as grasses and herbaceous species offering little structural diversity. Long fire intervals, in contrast, favour community development further along the successional trajectory, normally resulting in increased woody species with greater diversity.
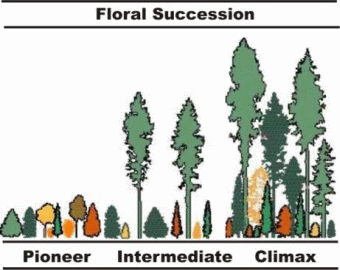
Figure 11. Floral succession from “pioneer” stage, through
“intermediate” stages, to a “climax” floral assemblage.
Faunal response
Faunal response closely mimics fire-induced changes to the floral succession of an ecosystem. Thus, fires that set succession back to a grass stage primarily benefit herbivores (vertebrates and invertebrates), and consequently, various vertebrate and invertebrate predators of herbivores are likely to also benefit. The loss of a single structural component, such as a feeding site, or nesting cover, can eliminate some faunal species, and can be just as lethal as direct fire mortality. Other important factors to consider are the interplay between one or more structural components; and the loss of mutually beneficial relationships between faunal species as a result of wildfire.
Species adaptability is of primary importance when evaluating the effect of fire on an ecosystem. Many studies have shown that animals that are broadly adaptable behaviourally and in their habitat preferences can accommodate change more efficiently, simply because they have more options (Dubos, 1972; Watt, 1972; Anderson, 2001). These species are frequently termed generalists, and are species of high versatility (Maser et al., 1984). At the opposite end of the spectrum are species that have a very narrow range of environmental conditions under which they can survive and flourish. These species of specialised adaptability are commonly termed specialists, and are species of low versatility (Maser et al., 1984). For example, an animal that depends on a comparatively small range of food items is more sensitive to fire effects than a species with more cosmopolitan dietary requirements. In other words, specialist species are less resilient in adapting to habitat modifications and are commonly eliminated by loss of a single habitat component.
As previously mentioned, no fire uniformly positively or negatively impacts an ecosystem, with some components flourishing from fire, and others decimated. This differential effect of fire is normally based on changes to food and cover/shelter, resulting in the realignment of the ecosystem as some species become favoured over others. Direct mortality effects are often thought of as insignificant; however, animals can be caught in the fires and die as a result. There are also indications that severe repetitive burning can cause long-term reductions in some insects and invertebrates of the soil litter and topsoil layers. In the long term these losses can have a significant effect on ecosystems.
There are a number of wildfire characteristics that directly influence faunal response. These include:
- Timing of the wildfire
- Size of burned area
- Burned area configuration
- Completeness of burn
Influence of time
Some species exhibit an almost immediate reaction to fire, while for others, behavioural time lags are common. In some instances, although the immediate damage to an ecosystem may look severe, generalist species are likely to accommodate change better than one might expect. A fire-damaged tree, for example, may not die for several years post-burn, and may be a sufficient new cavity for nesting birds. Timing is also important in relation to a plant’s stage of development. The size and stage of a tree bud, for example, influences its resistance to fire. The importance of timing is also highlighted in nesting birds, where the effect of fire can vary markedly depending on whether the fire occurred before, during, or after nesting activities were completed.
Burned area size
A number of smaller burns are likely to have less of a detrimental impact to an ecosystem compared with a single large burn.
Burned area configuration
Patchy or irregular burns have the ability to enhance habitat diversity, through making more niches available for partitioning (Anderson, 2001). Furthermore, the suitability of an area for individual species after the fire is governed by the areal extent, composition and orientation of habitat components such as food, cover, and water.
Completeness of burn
A low intensity fire which consumes comparatively little of the existing plant community may have little or no impact on wildlife. However, high severity fires have the ability to significantly alter habitat makeup, community succession and the structure of the ecosystem.
Indirect wildfire effects to wildlife
- Noxious weed invasion: any wildfire or prescribed fire occurring in an area leaves the area subject to noxious weed or exotic plant species invasion.
- Livestock use: livestock presence in an area following a fire can adversely affect an ecosystem. Post-fire sensitivity of an area, such as increased susceptibility to erosion, make control and management of livestock essential.
- Climatic events: climate and weather related phenomena such as abnormally high rainfall, unusually high or low temperatures, drought, and insect or disease outbreaks, are all factors that influence plant response time after a fire, and therefore indirectly affect wildlife in the area.
- Adverse effects to water quality: water quality can be adversely affected by the introduction of firefighting agents such as foam or fire retardants into surrounding waterways. Naturally, this will in turn have a major influence on food chain relationships.
Summary
Fire is obviously a shock to the ecological setting involved. Some fauna are able to adapt to the rapid change in environment, others “hang in there” for a little while, and some cannot. Fire effects are not black and white, the habitat of some species will be improved, while others will be degraded or completely destroyed, and there will be endless variation in between. How wildfire effects on ecosystems can be mitigated will be discussed in section five.
Effects on geosystems
Soil
It has historically been considered that the effect of fire on soil has always been negative, with fire impacting detrimentally on soil structure, soil nutrients and post-burn removal of the soil resource by fire-induced erosion. However, it has now been documented that these negative effects are often short-lived, and additionally, that occasionally fire (particularly if it is prescribed fire) has some beneficial effects on soil. Here we consider the impacts to the soil during the fire, inducing the physical effects, chemical effects, and the effects to soil biota and soil nutrients (Table 2).
Table 2: Changes in soil and plant material after heating (adapted from Walker, J., Raison, R. J. and Khanna, P. K. (1986))
Dominant type of change |
Temp °C |
Change |
|
>1200 |
Loss of calcium as gas |
Physical |
|
|
|
950 |
Clay minerals converted to different phases |
|
600 |
Maximum loss of potassium & phosphorus.
Fine ash produced |
|
540 |
Little residual nitrogen or carbon left |
|
420 |
Water lost from within clay minerals causing change in type |
|
400 |
Organic matter carbonized |
Chemical |
|
|
|
300 |
Maximum amino acid nitrogen released
Loss of sulphur and phosphorus begins
Organic matter charred |
|
200 |
Water repellance caused by distillation of volatiles
Loss of nitrogen commences |
|
125 |
Soil sterilization |
|
110 |
Soil water lost |
|
100 |
Soil ammonium production starts |
|
70 |
High nitrate mineralisation |
Biological |
|
|
|
50 |
Mild sterilization owing to water loss |
|
37 |
Maximum stimulation of soil microorganisms |
|
<25 |
Usual soil temperatures
|
a) During the fire
Soil heating
The magnitude of the heat pulse into the soil depends on fuel loading, fuel moisture content, fuel distribution, rate of combustion, soil texture, soil moisture content, and other factors (Clark, 2001). The penetration of heat into a mineral soil is dependant on both the peak temperature reached by the fire, and also the duration of time that the heat source is present. High intensity fires – those that reach 1200 ºC or more – do not always result in high severity impacts in the soil if their duration is short, but low intensity fires of just 300 ºC that smoulder for a long time in roots or organic matter can be very destructive. In addition, the pattern of soil heating varies greatly around a site and reflects the characteristics of the soil types involved. Highest soil temperatures (and greatest subsurface burning) are usually found beneath areas of greatest fuel consumption (particularly those with thick organic matter accumulations in the surface litter) and in the areas that have had the longest duration of burning.
Surface organic matter accumulation and soil moisture contents are important regulators of subsurface heating (Clark, 2001). Controlled heating experiments have revealed that surface temperatures are much higher when soils and soil surface litter is dry. In contrast, wet surface litter absorbs some of the heat transmitted downward into the soil, thus, resulting in less subsurface heating. Subsurface temperatures in “severely burned” areas have been known to approach 750ºC (Chandler et al., 1983). Little is known about the effects of heat on wetland soils. It is likely that due to the high water content of these soils, penetration of heat into the soil subsurface will be much less. Contrastingly, dry organic soils, such as peats, are likely to be much more flammable, particularly in drought situations, where subsurface fires can be hard to extinguish due to the hydrophobic nature of dry organic material (Fuller, 1991).
b) Post-fire temperature increases
The removal of the vegetative cover, as well as enhanced thermal effects from the ash cover, are likely to increase the temperature of the topsoil and subsurface soil after the fire event. After severe fires, soil surface temperatures have been known to rise as much as 5ºC as a result of black ash blanketing the soil surface, and the removal of the shade of trees (Fig. 12). This rise in soil temperature causes snow to melt earlier and in the longer term has a positive impact on plant growth.
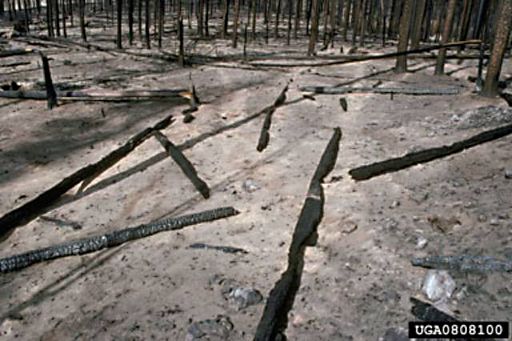
Figure 12. Grey ash blankets the soil surface post-fire
(Powell, Dave, USDA Forest Service, www.forestryimages.org).
c) Physical impacts on soil
Heating may cause changes in some physical properties of soils, including the loss or breakdown of soil structure, reduction of porosity, reduced moisture retention and capacity, and alteration of colour. Most frequently, however, the important consequences include the reduction of organic matter, the exhibition of hydrophobicity, and increased erosion due to the loss of protective plant and surface litter cover (Clark, 2001). Plant roots stabilise the soil, and stems and leaves slow the water to give it time to percolate into the soil profile. Moreover, when fire consumes vegetation and underlying litter layers, hydrophobic or water-repellent conditions can form.
The hydrophobic zone appears as a discrete layer in the soil, at or parallel to the surface, where hydrophobic organic compounds coat soil aggregates or minerals. This phenomenon occurs at soil temperatures of 176 to 288 ºC (DeBano, 1981). Hydrophobic soils prevent water from wetting aggregates, essentially sealing off the soil during rainfall, greatly increasing surface runoff and erosion. The net effect is a reduction in soil moisture content, and the erosion of nutrient-rich ash and upper soil horizon sediments. Drier soils are also detrimental to the viability of microbes that are involved in biogeochemical cycling and facilitate the recolonisation of plants that ultimately restabilise soils.
The severity and duration of accelerated erosion depends on several factors, including soil texture, slope, recovery time of protective cover, the amount of residual surface litter, and post-burn precipitation intensity. Furthermore, excessive erosion may not occur until a number of years after burning because root systems of burnt vegetation can maintain soil stability for a time.
d) Chemical impacts on soil
Certain chemical changes in soils may occur as the direct result of fire. These include a reduction of soil organic matter (and therefore nutrient reserves), an increase in pH, water repellent soil layers and hydrophobicity.
Organic matter
One of the most important impacts on soils results from the combustion of organic matter. Soil organic matter basically contains the nutrient reserves of a soil site. Organic matter is very important in many soil-ecosystem processes, including regulation of the hydrologic cycle, the C:N ratio, maintenance of soil structure, porosity and the cation exchange capacity (CEC) of a soil, and is the site for nitrogen-fixation by nitrogen-fixing bacteria. The amount of soil organic matter consumed in a fire relates to the pre-burn soil moisture content, and the amount and duration of heating. Campbell and others (1977) found that moderately burned areas maintained 40% of the vegetative and litter cover, whilst severely burned areas could lose between 75 and 100% of organic material. Furthermore, saturated soils rarely loose any organic matter, whereas substantial losses are common in dry and/or peaty soils.
pH
The combustion process releases bound nutrients which have the potential to increase soil pH. Certain cations (positive ions) are stable at typical combustion temperatures, and remain onsite after burning, in the form of ash or uncombusted hydrocarbons. These cations are subsequently leached into the soil where they exchange with H+ ions; the resulting increase in H+ ions in solution lowers the pH (or increases the acidity) (Clark, 2001). Nutrient availability is related to soil acidity, with nutrients critical for plant growth, such as nitrogen and phosphorus, becoming increasingly available with increases in soil pH.
Soil nutrients
Soil nutrient changes certainly occur during the combustion process. When fuel burns, a large amount of nutrients remain on the site in the ash (converted into a different more available form), some may leave the site via overland flow, or may volatilise and leave the site in gaseous form (Clark, 2001). Nitrogen, phosphorus, potassium, sulphur, and calcium can vaporise in a severe fire. In the former case, nitrogen volatilises at very low temperatures, yet although the total site nitrogen budget decreases, usually the ammonium (NH4+) form increases (Fuller, 1991). Moreover, following the first significant post-fire rain event, nitrogen is frequently lost through leaching by water percolating into the lower layers of the soil. Counteracting this loss, the ash from burned fuel adds minerals, like potassium to the soil; the “ash effect”, acting as a fertiliser.
Recovery of soil nutrient levels after fires can be fairly slow in some ecosystems, particularly those with limited nitrogen, and in semi-arid regions where decomposition rates are slow. A study by Klopatek (1987) for example, determined that 35 years after a wildfire, nitrogen concentrations in pinyon-juniper woodland soils in the United States had not recovered to levels found in nearby soils that had not been burned for 300 years.
e) Soil biology
The short- and long-term effects of fire on soil micro-organisms, and the resulting effects on ecosystem sustainability, are uncertain and often debated. Soil fauna are variously affected by wildfire and prescribed fires. Aboveground, soil-related herbivores and carnivores will suffer with temporary declines. Sub-surface biota respond differently depending on the duration and degree of soil heating (fire intensity), the site conditions pre- and post-fire, and the specific organism in question.
Low severity, rapid moving fires do not have a major effect on microbial populations, whereas, high severity fires with long durations have the greatest impact. Field studies by Klopatek and others (1988), for example, showed that ten years after burning, mycorrhizae fungi, soil nutrients, and vegetation with severely burned canopies had not recovered to pre-burn levels.
The amount of moisture in the soil when the fire occurs is a critical factor in terms of biota mortality. Water in soil increases the rate of conductance so that elevated subsoil temperatures are reached more quickly in drier and often coarser soils.
Fire-induced changes in the soil environment may favour one soil micro-organism to the detriment of another. Mycorrhizal fungi (Fig. 13), for instance, are known to form a symbiotic relationship with the roots of most higher plant species. These fungal strands are responsible for absorbing water and nutrients (particularly phosphorus) from the soil and translocating it to the roots of the host plant. The host plant in return provides photosynthetic products to the fungi. The presence of these fungi can be critical to the establishment of certain plant species. Most mycorrhizal roots occur in the surface horizons and organic surface layer, thus, if fire removes most of the organic matter on a forested site, productivity can be significantly reduced for many years.
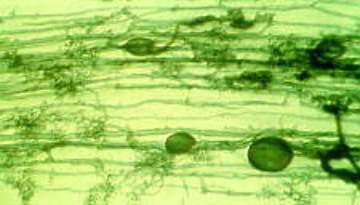
Figure 13. Mycorrhizal fungi. The role of this complex network of fungal
hyphae is to translocate nutrients to the roots of the host plant
(photo courtesy of Brundrett, M, www.mycolog.com).
Water quality
Wild and prescribed fires on forestlands, shrublands and grasslands have the potential to decrease on and off-site water quality. Fire-induced changes in water quality include increased sedimentation and turbidity, increased stream temperatures, and increased concentrations of nutrients resulting from surface runoff.
- w Sedimentation and turbidity reduce the quality of spawning areas in river systems, and reduce photosynthetic activity (Clark, 2001).
- w Addition of sediment and nutrients following fire (increased erosion and sediment runoff into waterways) may reduce the oxygen content below acceptable levels, adversely impacting fisheries.
- w Stream temperatures can increase after wildfire due to the removal of overhead protective vegetation, and are likely to be detrimental to most cold water fish species.
- w Increased nutrient runoff into waterways can result in elevated levels of bicarbonates, nitrates, ammonium, and organic nitrogen, and are likely to contribute to stream eutrophication or algal blooms (Fig. 14) (Chandler et al., 1983).
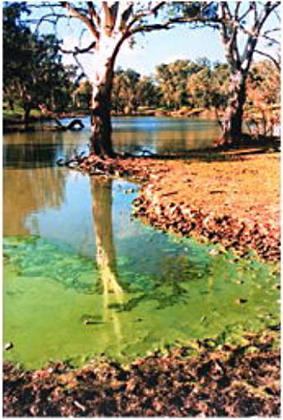
Figure 14. Stream eutrophication
(photo courtesy of the Australian Government-Land and Water Australia, www.rivers.gov.au).
It should also be noted that the use of fire retardant chemicals to combat wildfires may be toxic to aquatic animals, and use of these chemicals should be avoided near or in water courses.
Wildfires followed by intense rainstorms can be extremely damaging, resulting in massive flooding and overland flows of sediment, as well as scouring of creek channels and deposition of soil and debris in rivers and reservoirs. Large, high-severity wildfires pose a much greater threat to water quality than do low-severity wild or prescribed fires (Omi, 2005).
The effects of fire on soils, water and watersheds are extremely variable. In cases, of accelerated erosion, the outcome is reasonably predictable and mitigating measures such as rapid revegetation are necessary. In other cases, the outcome is much less predictable because it depends on site-specific characteristics and on unpredictable climatic events. The application of mitigation measures must therefore be based on local experience and local research.
Effects on the atmosphere
Fire has been affecting the Earth’s atmosphere since the emergence of land plants through the ignition of wildfires by lightning. More recently, humans have become the main factor in fires, through processes such as forest clearance and burning to sustain nomadic agriculture. The rapid rise in human population has led to major changes in fires (e.g., sites, size, and frequency), with consequences for atmospheric conditions and climate. Of concern to Earth scientists is the impact widespread forest fires and wildfires have on climate. Fire releases huge amounts of smoke, carbon dioxide, and methane into the atmosphere. These emission products promote the formation of polluted clouds and affect the Earth’s radiant energy budget (heat and sunlight), acting like “insulation” in the atmosphere, trapping and retaining heat emitted from the surface. Such changes to the natural radiant energy budget is thought to influence climate on both a regional and global scale, and is likely to contribute to global warming (Christopher, 1996).
This section will detail the emission products of the combustion process and their effect on the atmosphere, the fuel properties that affect smoke production, the human health risks from smoke, as well as an introduction to satellite remote sensing techniques that are used to monitor biomass burning and smoke aerosol yield during wildfire events.
Combustion products
When wood burns in a completely efficient manner, it combines with atmospheric oxygen, and produces water, carbon dioxide, and energy. However, the combustion of fuels during wildfires is not a completely efficient process. The most important reason for incomplete combustion is that wind cannot deliver enough oxygen to the combustion zone to mix efficiently with all of the flammable gas produced (Mahaffey and Miller, 2001). Hundreds of different compounds are emitted in the smoke from wildfires, however, visible smoke consists mainly of tar, soot, and water vapour. Wildfire emissions are dependant on which phase of the four phase combustion process is occurring. The four phases of combustion are as follows: pre-ignition, flaming, smouldering, and the glowing phase.
During the pre-ignition phase gases, vapours, tars, and charcoals are produced. The proportions and amounts vary widely according to the conditions under which pyrolysis occurs (Mahaffey and Miller, 2001). If rapid heating occurs less charcoal, a lot of tar, and highly flammable gases are produced. Slow heating during pyrolysis results in the production of more charcoal, little tar, and lower amounts of flammable gases.
The principal chemical by-products of flaming combustion are carbon dioxide and water. However, where substances are only partially oxidized, tar droplets and particles of soot can also result.
The lower temperatures of the smouldering phase allow some gases to cool and condense as visible smoke (Mahaffey and Miller, 2001). Smouldering fires produce at least twice as many emissions as flaming fires. Fire heat is inadequate to loft the smoke as a convection column, so smoke often stays very near to the ground.
During the glowing phase of the combustion process combustion is fairly efficient with carbon monoxide and carbon dioxide dominating the emission products. No visible smoke is usually formed during this phase.
Fuel properties affecting smoke production
Fuel properties that affect the volume of smoke produced include: particle size, arrangement and continuity, fuel loading, and fuel moisture.
Fuel particle size, arrangement, and continuity
As previously mentioned, the smaller the size of the particle, the higher the surface area to volume ratio, and therefore, the more quickly the fuel is likely to ignite and be consumed. The arrangement of fuel particles influences the amount of oxygen that reaches them. Therefore, the more tightly packed the fuel, the less efficiently it burns, and subsequently, the more smoke it produces. Fuel continuity is also an issue as sustained ignition cannot occur if fuel is too widely spaced.
Fuel loading
The size class distribution of the fuel is important as the proportion of fuel in each size affects the proportion that may be consumed in flaming versus smouldering combustion. Smaller sized fuels, for example, such as loosely packed grass litter, burn almost entirely in flames with little residual smouldering (i.e. less smoke produced). In contrast, large diameter woody fuels, such as logs, are rarely consumed in flaming combustion, and thus have a higher potential to emit large amounts of smoke (Mahaffey and Miller, 2001).
Fuel moisture
The moisture content of fuel affects smoke production because it influences the temperature at which combustion occurs. Extremely dry fuels burn rapidly and completely, whilst wet fuels burn slowly and often incompletely. If larger fuels have a high moisture content, most or all of the heat released by flaming combustion will be expended evaporating the water, thus, little consumption of the fuel occurs. Furthermore, the little that does is likely to be incomplete with large amounts of smoke.
Human health risks from smoke
There is a growing concern that smoke from wildfires can expose individuals and populations to hazardous air pollutants. Concern is increasing over the risk to firefighters and the general public from exposure to toxins, irritants, and known carcinogens in smoke (see additional readings 4 (1) and (2)). Firefighters can be exposed to high levels of lung toxins such as aldehydes, acids, and particulates; to carcinogens such as polycyclic aromatic hydrocarbons, formaldehyde and benzene; and to carbon monoxide.
By definition, smoke inhalation injury is the aspiration of superheated gases, steam, hot liquids or noxious products of incomplete combustion that cause thermal or chemical injury to the airways and lungs (Peck, 2005). The inhalation of super-heated air or gases may cause rapid swelling of the throat which can compress the windpipe and cause asphyxiation. Smoke inhalation can cause asphyxiation. Smoke is not a gas, it is composed of particles of carbon from burnt materials which are light enough to float in the air and so small as not to appear as ash. Smoke particles can be as small as 0.01 of a micron in diameter. The lungs are able to expel large particles but those as small as smoke particles lodge themselves in the fine passages of the bronchi and become trapped. Clogging the airways with smoke particles can cause difficulty breathing, pneumonias or chronic lung inflammations, and in the worse case death.
Contrary to popular opinion, lack of oxygen is not a killer factor during a wildfire. It is true that wildfires consume a lot of oxygen, however, if all the oxygen were sucked out of the air, the fire would go out, as oxygen is essential for combustion. Carbon dioxide does not affect people during a wildfire. Rather, it is in closed spaces that carbon monoxide has caused fire deaths. Other poisonous gases may be given off from ignited materials and may affect humans, again, if they are in an enclosed space.
Although there is a low probability that public health is at risk during a wildfire event, it is possible that firefighters can be exposed to high levels of lung toxins, depending upon the concentration, duration, and level of activity of the firefighters at the time of exposure. Carbon monoxide is a chemical asphyxiant that interferes with oxygen transport in blood. Firefighters exposed to carbon monoxide have developed headaches, fatigue, decreased concentration and impaired judgement. Data also suggests that long-term exposure to low levels of carbon monoxide produces accelerated arteriosclerosis, increasing the risk of cardiovascular diseases such as heart attack and stroke (Mahaffey and Miller, 2001).
Formaldehyde is a very common atmospheric contaminant, produced in association with building material, textiles, and has also been measured as a by-product of burning wood. Firefighters may experience nose, throat, and eye irritation, when exposed to low levels of formaldehyde, whereas at higher concentrations it may interfere with their ability to obtain adequate oxygen. Acrolein, another aldehyde, is also a by-product of wildfire burning, with studies suggesting that greater amounts of this product is produced in inefficient fires (Mahaffey and Miller, 2001). It has similar effects as formaldehyde to the respiratory system, but may also have severe toxic effects on cells.
PAHs or Polynuclear aromatic hydrocarbons are known by-products of the burning of wood, some of which have carcinogenic properties. The likely risk of the general public developing cancer is very low, however, very little is known about the potential effects on firefighters.
Smoke and emission monitoring
There are several approaches that can be used to monitor smoke and other wildfire emissions. Prior to the use of remote sensing satellites, aircraft tracking of smoke plumes was used to verify the source and trajectory of the smoke. More recently, satellite remote sensing gives a global view of several parameters related to biomass burning, monitoring the fires themselves, smoke aerosols, and the burnt area after the event. Using this technique, seasonal distributions and inter-annual variability of biomass burning can be obtained, and mitigation and preventative measure put into place. These techniques will be discussed in section Satellite monitoring.
Nephelometers and filter samplers can be used to measure certain parameters relating to the amount of particulate matter a wildfire is producing. A nephelometer is a device that measures the amount of particulates in a sample of air. It is an optical device that uses the light reflected from particles in an enclosed sample space to estimate the amount of smoke in the air. Such an instrument can be used as part of a warning system on a highway, triggering flashing lights when smoke-induced visibility is poor. Another useful apparatus is the filter sampler which can be used to calculate the weight of particulate per volume of air. This equipment works by drawing a known volume of air through a filter, the filter being weighed both before and after the sampling period, so that the weight of particulate can be established.
Effects on Society
Every society has its own unique set of archaeological and architectural remains resulting from human activity in the prehistoric and historic period. These cultural resources can include archaeological sites such as stone tool quarries, artefact scatters, and rock art locations, through to battlegrounds, early mining remains, and ceremonial sites, valued for religious purposes. These are all highly valued sites containing a treasury of important historical information that must be preserved for the coming generations. The contribution of such sites to the recreational economy of some areas is significant as well. However, like any location, given the right conditions, wildfire can be a serious threat to the preservation of these important cultural resources. Prehistorically, it is likely that many of these sites have been naturally exposed to fire, perhaps frequently. However, given the past fire suppression policies of many countries, wildfires occurring around these sites have the potential to have greater impact due to increased fuel accumulation, and consequently the increased amounts of heat that can be released during burning.
Literature addressing the effects of fire and heat on archaeological remains is rather scant; however, enough information exists to indicate that the effects are variable, and depend on the nature of the material that is being heated, whether it is buried or on the surface, the intensity, and the duration of heating. Most subsurface artefacts are insulated from the heat of the fire by the earth, and depending on aboveground fuel load and the soil moisture content of the soil, may not be affected by a raging wildfire. However, aboveground structures, such as old buildings, will naturally be at greater risk.
Physical damage to materials
a) Stone
Damage is governed primarily on the temperature reached during heating. Laboratory experiments have demonstrated that the crystalline structure of many forms of silica-rich stone changes when heated above 370º C (700º F) (Purdy and Brooks, 1971). Beyond that temperature, stone will shatter, crack, or oxidise (often a colour change) from direct exposure to heat. Rock art sites, such as pictographs (painted stone) practised by the Australian aborigines, or petroglyphs (stone carvings) common to the Moriori of the Chatham Islands, are especially susceptible to fire damage. When exposed to intense heat, paintings can be scorched or completely burned away, whilst, depending on the petroglyph substrate, fire can cause exfoliation (usually affecting limestone or sandstone carvings).
b) Organics
Artefacts constructed of organic materials such as woven baskets, wooden tools, and fur clothing, are very fragile and susceptible to complete consumption at low temperatures. Shell and bone are common to many archaeological sites, often giving evidence about the nature of the diet, or ceremonial attire. When subjected to high temperatures shell and bone can become brittle and friable. Pollen grains, which are often used in paleoenvironmental reconstructions and dietary studies, are destroyed at temperatures greater than 300º C (Traylor, 1981).
c) Ceramics, metals, and glass
Pottery is made by subjecting worked pieces to intense heat. Wildfires of long duration and high intensity have the ability to re-fire ceramic works, often resulting in increased brittleness. Heating can also affect the chemical composition of glazed or painted ceramics, resulting in colour changes and the deposition of carbon on the surface of the artefacts. Metals and glass have the ability to fuse or melt together given high enough heating.
d) Effects on archaeological dating techniques
Tree-ring (Fig. 15), radiocarbon, thermoluminescene, obsidian hydration, and cation ratio dating, can all be used to derive absolute or relative dates of site occupation. These methods can also be adversely affected by wildfire, giving erroneous information and more often than not significantly younger dates than the true date of the sample.
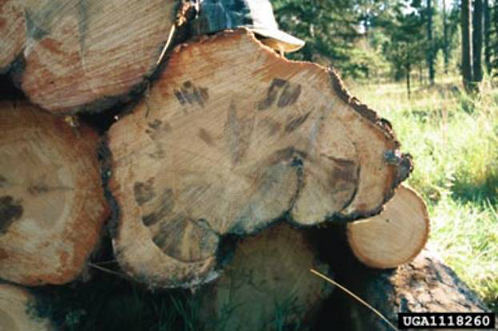
Figure 15. Fire scars on a cross-sectioned ponderosa tree trunk
(at least 2 separate events are visible here)
(Lockhart, Brian, USDA Forest Service, www.forestryimages.org).
e) Indirect impacts
Fire management/mitigation techniques
The use of mechanical equipment, fire suppression, and rehabilitation operations,
often have the most dramatic impacts on cultural resources, frequently having a greater effect on archaeological sites than did the actual fire itself. Yet impacts from these activities are also the most preventable. In all clean-up and fire prevention instances planning, and coordination with archaeologists familiar with the site is crucial in the preservation of cultural resources. In recent years, loss of ground cover, and enhanced visibility of archaeological sites, has led to the illegal collection and excavation of archaeological material. This may need to be taken into consideration, and reasonable measures put in place.
Summary
Damage to cultural resources posed by wildfires can be severe, ranging from chemical alteration to exfoliation of rock art panels, to total consumption of wooden tools. However, most impacts can be avoided through advanced planning. Knowing the fire regime of the ecosystem you are managing – the vegetation, climate, and terrain – is key to understanding the behaviour of fire. Furthermore, fire management techniques in these high risk cultural areas could include removal of high fuel loads by hand or prescribed fire and/or the use of firebreaks around wooden structures and the like. These mitigation measures will be examined further in the next section.
Impacts of Wildfires – Readings
Read the following two reprints complementing the study guide text.
(1) Coghlan, B. (2004). The human health impact of the 2001-2002 ‘Black Christmas’ bushfires in New South Wales, Australia: an alternative multidisciplinary strategy. Journal of Rural and Remote Sensing Environmental Health 3 (1): 18-28.www.jcu.edu.au/jrtph/vol/v03coghlan.pdf
(2) Peck, M. (2005). Smoke inhalation injury. www.nfrmag.com/Sept%20Oct%2005/Smoke.asp
|
|